Applied NMR Spectroscopy for Chemists and Life Scientists by Oliver Zerbe, Simon Jurt
Applied NMR Spectroscopy for Chemists and Life Scientists
From complex structure elucidation to biomolecular interactions - this applicationoriented textbook covers both theory and practice of modern NMR applications.
Part one sets the stage with a general description of NMR introducing important parameters such as the chemical shift and scalar or dipolar couplings. Part two describes the theory behind NMR, providing a profound understanding of the involved spin physics, deliberately kept shorter than in other NMR textbooks, and without a rigorous mathematical treatment of all the physico-chemical computations. Part three discusses technical and practical aspects of how to use NMR. Important phenomena such as relaxation, exchange, or the nuclear Overhauser effects and the methods of modern NMR spectroscopy including multidimensional experiments, solid state NMR, and the measurement of molecular interactions are the subject of part four. The final part explains the use of NMR for the structure determination of selected classes of complex biomolecules, from steroids to peptides or proteins, nucleic acids, and carbohydrates.
For chemists as well as users of NMR technology in the biological sciences.
Preface XV
1 Introduction to NMR Spectroscopy 1
1.1 Our First 1D Spectrum 1
1.2 Some Nomenclature: Chemical Shifts, LineWidths, and Scalar Couplings 2
1.3 Interpretation of Spectra: A Simple Example 5
1.4 Two-Dimensional NMR Spectroscopy: An Introduction 9
Part One Basics of Solution NMR 11
2 Basicsof1DNMRSpectroscopy 13
2.1 The Principles of NMR Spectroscopy 13
2.2 The Chemical Shift 16
2.3 Scalar Couplings 17
2.4 Relaxation and the Nuclear Overhauser Effect 20
2.5 Practical Aspects 23
2.5.1 Sample Preparation 23
2.5.2 Referencing 25
2.5.3 Sensitivity and Accumulation of Spectra 27
2.5.4 Temperature Calibration 29
2.6 Problems 30
Further Reading 31
3 1H NMR 33
3.1 General Aspects 33
3.2 Chemical Shifts 34
3.2.1 Influence of Electronegativity of Substituents 35
3.2.2 Anisotropy Effects 35
3.2.3 Other Factors Affecting Chemical Shifts: Solvent, Temperature, pH, and Hydrogen Bonding 37
3.2.4 Shift Reagents 37
3.3 Spin Systems, Symmetry, and Chemical or Magnetic Equivalence 39
3.3.1 Homotopic, Enantiotopic, and Diastereotopic Protons 42
3.3.2 Determination of Enantiomeric Purity 43
3.4 Scalar Coupling 44
3.4.1 First-Order Spectra 45
3.4.2 Higher-Order Spectra and Chemical Shift Separation 47
3.4.3 Higher-Order Spectra and Magnetic Equivalence 49
3.5 1H–1H Coupling Constants 50
3.5.1 Geminal Couplings 50
3.5.2 Vicinal Couplings 50
3.5.3 Long-Range Couplings 52
3.5.4 1HCouplings to Other Nuclei 52
3.6 Problems 54
Further Reading 55
4 NMRof13C and Heteronuclei 57
4.1 Properties of Heteronuclei 57
4.2 Indirect Detection of Spin-1/2 Nuclei 59
4.3 13C NMR Spectroscopy 59
4.3.1 The 13C Chemical Shift 60
4.3.2 X,13C Scalar Couplings 64
4.3.3 Longitudinal Relaxation of 13C Nuclei 68
4.3.4 Recording 13C NMR Spectra 68
4.4 NMR of Other Main Group Elements 70
4.4.1 Main Group Nuclei with I D 1/2 71
4.4.2 Main Group Nuclei with I > 1/2 75
4.5 NMR Experiments with Transition Metal Nuclei 78
4.5.1 Technical Aspects of Inverse Experiments with I D 1/2 Metal Nuclei 79
4.5.2 Experiments with Spin I > 1/2 Transition Metal Nuclei 81
4.6 Problems 82
Further Reading 84
Part Two Theory of NMR Spectroscopy 85
5 Nuclear Magnetism – A Microscopic View 87
5.1 The Origin of Magnetism 87
5.2 Spin – An Intrinsic Property of Many Particles 88
5.3 Experimental Evidence for the Quantization of the Dipole Moment: The Stern–Gerlach Experiment 93
5.4 The Nuclear Spin and Its Magnetic Dipole Moment 94
5.5 Nuclear Dipole Moments in a Homogeneous Magnetic Field: The Zeeman Effect 96
5.5.1 Spin Precession 98
5.6 Problems 103
6 Magnetization – A Macroscopic View 105
6.1 The Macroscopic Magnetization 105
6.2 Magnetization at Thermal Equilibrium 106
6.3 Transverse Magnetization and Coherences 108
6.4 Time Evolution of Magnetization 109
6.4.1 The Bloch Equations 110
6.4.2 Longitudinal and Transverse Relaxation 112
6.5 The Rotating Frame of Reference 115
6.6 RF Pulses 117
6.6.1 Decomposition of the RF Field 118
6.6.2 Magnetic Fields in the Rotating Frame 119
6.6.3 The Bloch Equations in the Rotating Frame 120
6.6.4 Rotation of On-Resonant and Off-Resonant Magnetization under the Influence of Pulses 121
6.7 Problems 122
7 Chemical Shift and Scalar and Dipolar Couplings 125
7.1 Chemical Shielding 125
7.1.1 The Contributions to Shielding 127
7.1.2 The Chemical Shifts of Paramagnetic Compounds 131
7.1.3 The Shielding Tensor 132
7.2 The Spin–Spin Coupling 133
7.2.1 Scalar Coupling 134
7.2.2 Quadrupolar Coupling 140
7.2.3 Dipolar Coupling 141
7.3 Problems 144
Further Reading 145
8 A Formal Description of NMR Experiments: The Product Operator Formalism 147
8.1 Description of Events by Product Operators 148
8.2 Classification of Spin Terms Used in the POF 149
8.3 Coherence Transfer Steps 151
8.4 An Example Calculation for a Simple 1D Experiment 152
Further Reading 153
9 A Brief Introduction into the Quantum-Mechanical Concepts of NMR 155
9.1 Wave Functions, Operators, and Probabilities 155
9.1.1 Eigenstates and Superposition States 156
9.1.2 Observables of Quantum-Mechanical Systems and Their Measured Quantities 157
9.2 Mathematical Tools in the Quantum Description of NMR 158
9.2.1 Vector Spaces, Bra’s, Ket’s, and Matrices 158
9.2.2 Dirac’s Bra–Ket Notation 159
9.2.3 Matrix Representation of State Vectors 160
9.2.4 Rotations between State Vectors can be Accomplished with Tensors 161
9.2.5 Projection Operators 162
9.2.6 Operators in the Bra–Ket Notation 163
9.2.7 Matrix Representations of Operators 165
9.3 The Spin Space of Single Noninteracting Spins 166
9.3.1 Expectation Values of the Spin-Components 168
9.4 Hamiltonian and Time Evolution 169
9.5 Free Precession 169
9.6 Representation of Spin Ensembles – The Density Matrix Formalism 171
9.6.1 Density Matrix at Thermal Equilibrium 173
9.6.2 Time Evolution of the Density Operator 173
9.7 Spin Systems 175
9.7.1 Scalar Coupling 176
Part Three Technical Aspects of NMR 179
10 The Components of an NMR Spectrometer 181
10.1 The Magnet 181
10.1.1 Field Homogeneity 182
10.1.2 Safety Notes 183
10.2 Shim System and Shimming 184
10.2.1 The Shims 184
10.2.2 Manual Shimming 185
10.2.3 Automatic Shimming 186
10.2.4 Using Shim Files 187
10.2.5 Sample Spinning 187
10.3 The Electronics 187
10.3.1 The RF Section 188
10.3.2 The Receiver Section 189
10.3.3 Other Electronics 189
10.4 The Probehead 189
10.4.1 Tuning and Matching 190
10.4.2 Inner and Outer Coils 191
10.4.3 Cryogenically Cooled Probes 191
10.5 The Lock System 192
10.5.1 The 2H Lock 192
10.5.2 Activating the Lock 193
10.5.3 Lock Parameters 194
10.6 Problems 194
Further Reading 194
11 Acquisition and Processing 195
11.1 The Time Domain Signal 197
11.2 Fourier Transform 199
11.2.1 Fourier Transform of Damped Oscillations 199
11.2.2 Intensity, Integral, and Line Width 200
11.2.3 Phases of Signals 201
11.2.4 Truncation 202
11.2.5 Handling Multiple Frequencies 202
11.2.6 Discrete Fourier Transform 203
11.2.7 Sampling Rate and Aliasing 204
11.2.8 How Fourier Transformation Works 205
11.3 Technical Details of Data Acquisition 209
11.3.1 Detection of the FID 209
11.3.2 Simultaneous and Sequential Sampling 210
11.3.3 Digitizer Resolution 213
11.3.4 Receiver Gain 214
11.3.5 Analog and Digital Filters 215
11.3.6 Spectral Resolution 216
11.4 Data Processing 217
11.4.1 Digital Resolution and Zero Filling 217
11.4.2 Linear Prediction 219
11.4.3 Pretreatment of the FID: Window Multiplication 220
11.4.4 Phase Correction 227
11.4.5 Magnitude Mode and Power Spectra 229
11.4.6 Baseline Correction 230
11.5 Problems 231
Further Reading 232
12 Experimental Techniques 233
12.1 RF Pulses 233
12.1.1 General Considerations 234
12.1.2 Hard Pulses 235
12.1.3 Soft Pulses 236
12.1.4 Band-Selective RF Pulses 237
12.1.5 Adiabatic RF Pulses 238
12.1.6 Composite Pulses 240
12.1.7 Technical Considerations 241
12.1.8 Sources and Consequences of Pulse Imperfections 243
12.1.9 RF Pulse Calibration 244
12.1.10 Transmitter Pulse Calibration 245
12.1.11 Decoupler Pulse Calibration (13C and 15N) 246
12.2 Pulsed Field Gradients 247
12.2.1 Field Gradients 247
12.2.2 Using Gradient Pulses 248
12.2.3 Technical Aspects 250
12.3 Phase Cycling 251
12.3.1 The Meaning of Phase Cycling 251
12.4 Decoupling 255
12.4.1 How Decoupling Works 255
12.4.2 Composite Pulse Decoupling 256
12.5 Isotropic Mixing 257
12.6 Solvent Suppression 257
12.6.1 Presaturation 258
12.6.2 Water Suppression through Gradient-Tailored Excitation 259
12.6.3 Excitation Sculpting 260
12.6.4 WET 260
12.6.5 One-Dimensional NOESY with Presaturation 260
12.6.6 Other Methods 261
12.7 Basic 1D Experiments 262
12.8 Measuring Relaxation Times 262
12.8.1 Measuring T1 Relaxation – The Inversion-Recovery Experiment 262
12.8.2 Measuring T2 Relaxation – The Spin Echo 263
12.9 The INEPT Experiment 266
12.10 The DEPT Experiment 268
12.11 Problems 270
13 The Art of Pulse Experiments 271
13.1 Introduction 271
13.2 Our Toolbox: Pulses, Delays, and Pulsed Field Gradients 272
13.3 The Excitation Block 273
13.3.1 A Simple 90ý Pulse Experiment 273
13.3.2 The Effects of 180ý Pulses 273
13.3.3 Handling of Solvent Signals 274
13.3.4 A Polarization Transfer Sequence 275
13.4 The Mixing Period 277
13.5 Simple Homonuclear 2D Sequences 278
13.6 Heteronuclear 2D Correlation Experiments 279
13.7 Experiments for Measuring Relaxation Times 281
13.8 Triple-Resonance NMR Experiments 283
13.9 Experimental Details 284
13.9.1 Selecting the Proper Coherence Pathway: Phase Cycles 284
13.9.2 Pulsed Field Gradients 286
13.9.3 N-Dimensional NMR and Sensitivity Enhancement Schemes 288
13.10 Problems 289
Further Reading 289
Part Four Important Phenomena and Methods in Modern NMR 291
14 Relaxation 293
14.1 Introduction 293
14.2 Relaxation: The Macroscopic Picture 293
14.3 The Microscopic Picture: Relaxation Mechanisms 294
14.3.1 Dipole–Dipole Relaxation 295
14.3.2 Chemical Shift Anisotropy 297
14.3.3 Scalar Relaxation 298
14.3.4 Quadrupolar Relaxation 298
14.3.5 Spin–Spin Rotation Relaxation 299
14.3.6 Paramagnetic Relaxation 299
14.4 Relaxation and Motion 299
14.4.1 A Mathematical Description of Motion: The Spectral Density Function 300
14.4.2 NMR Transitions That Can Be Used for Relaxation 302
14.4.3 The Mechanisms of T1 and T2 Relaxation 303
14.4.4 Transition Probabilities 304
14.4.5 Measuring Relaxation Rates 306
14.5 Measuring 15N Relaxation to Determine Protein Dynamics 306
14.5.1 The Lipari–Szabo Formalism 307
14.6 Measurement of Relaxation Dispersion 310
14.7 Problems 313
15 The Nuclear Overhauser Effect 315
15.1 Introduction 315
15.1.1 Steady-State and Transient NOEs 318
15.2 The Formal Description of the NOE: The Solomon Equations 318
15.2.1 Different Regimes and the Sign of the NOE: Extreme Narrowing and Spin Diffusion 320
15.2.2 The Steady-State NOE 321
15.2.3 The Transient NOE 324
15.2.4 The Kinetics of the NOE 324
15.2.5 The 2D NOESY Experiment 325
15.2.6 The Rotating-Frame NOE 327
15.2.7 The Heteronuclear NOE and the HOESY Experiment 329
15.3 Applications of the NOE in Stereochemical Analysis 330
15.4 Practical Tips for Measuring NOEs 332
15.5 Problems 333
Further Reading 334
16 Chemical and Conformational Exchange 335
16.1 Two-Site Exchange 335
16.1.1 Fast Exchange 338
16.1.2 Slow Exchange 340
16.1.3 Intermediate Exchange 340
16.1.4 Examples 342
16.2 Experimental Determination of the Rate Constants 344
16.3 Determination of the Activation Energy by Variable-Temperature NMR Experiments 346
16.4 Problems 348
Further Reading 349
17 Two-Dimensional NMR Spectroscopy 351
17.1 Introduction 351
17.2 The Appearance of 2D Spectra 352
17.3 Two-Dimensional NMR Spectroscopy: How Does It Work? 354
17.4 Types of 2D NMR Experiments 357
17.4.1 The COSY Experiment 358
17.4.2 The TOCSY Experiment 359
17.4.3 The NOESY Experiment 362
17.4.4 HSQC and HMQC Experiments 364
17.4.5 The HMBC Experiment 365
17.4.6 The HSQC-TOCSY Experiment 366
17.4.7 The INADEQUATE Experiment 367
17.4.8 J-Resolved NMR Experiments 368
17.5 Three-Dimensional NMR Spectroscopy 370
17.6 Practical Aspects of Measuring 2D Spectra 370
17.6.1 Frequency Discrimination in the Indirect Dimension: Quadrature Detection 370
17.6.2 Folding in 2D Spectra 376
17.6.3 Resolution in the Two Frequency Domains 377
17.6.4 Sensitivity of 2D NMR Experiments 378
17.6.5 Setting Up 2D Experiments 379
17.6.6 Processing 2D Spectra 380
17.7 Problems 381
18 Solid-State NMR Experiments 383
18.1 Introduction 383
18.2 The Chemical Shift in the Solid State 384
18.3 Dipolar Couplings in the Solid State 386
18.4 Removing CSA and Dipolar Couplings: Magic-Angle Spinning 387
18.5 Reintroducing Dipolar Couplings under MAS Conditions 388
18.5.1 An Alternative to Rotor-Synchronized RF Pulses: Rotational Resonance 390
18.6 Polarization Transfer in the Solid State: Cross-Polarization 391
18.7 Technical Aspects of Solid-State NMR Experiments 393
18.8 Problems 394
Further Reading 394
19 Detection of Intermolecular Interactions 395
19.1 Introduction 395
19.2 Chemical Shift Perturbation 397
19.3 Methods Based on Changes in Transverse Relaxation (Ligand-Observe Methods) 398
19.4 Methods Based on Changes in Cross-Relaxation (NOEs) (Ligand-Observe or Target-Observe Methods) 400
19.5 Methods Based on Changes in Diffusion Rates (Ligand-Observe Methods) 403
19.6 Comparison of Methods 404
19.7 Problems 405
Further Reading 406
Part Five Structure Determination of Natural Products by NMR 407
20 Carbohydrates 419
20.1 The Chemical Nature of Carbohydrates 419
20.1.1 Conformations of Monosaccharides 422
20.2 NMR Spectroscopy of Carbohydrates 423
20.2.1 Chemical Shift Ranges 423
20.2.2 Systematic Identification by NMR Spectroscopy 424
20.2.3 Practical Tips: The Choice of Solvent 429
20.3 Quick Identification 430
20.4 A Worked Example: Sucrose 430
Further Reading 437
21 Steroids 439
21.1 Introduction 439
21.1.1 The Chemical Nature 440
21.1.2 Proton NMR Spectra of Steroids 441
21.1.3 Carbon Chemical Shifts 443
21.1.4 Assignment Strategies 444
21.1.5 Identification of the Stereochemistry 447
21.2 A Worked Example: Prednisone 449
Further Reading 456
22 Peptides and Proteins 457
22.1 Introduction 457
22.2 The Structure of Peptides and Proteins 458
22.3 NMR of Peptides and Proteins 461
22.3.1 1HNMR 461
22.3.2 13C NMR 464
22.3.3 15N NMR 467
22.4 Assignment of Peptide and Protein Resonances 469
22.4.1 Peptides 470
22.4.2 Proteins 473
22.5 A Worked Example: The Pentapeptide TP5 476
Further Reading 480
23 Nucleic Acids 481
23.1 Introduction 481
23.2 The Structure of DNA and RNA 482
23.3 NMR of DNA and RNA 486
23.3.1 1HNMR 486
23.3.2 13C NMR 489
23.3.3 15NNMR 490
23.3.4 31P NMR 490
23.4 Assignment of DNA and RNA Resonances 492
23.4.1 Unlabeled DNA/RNA 492
23.4.2 Labeled DNA/RNA 496
Further Reading 498
Appendix 499
A.1 The Magnetic H and B Fields 499
A.2 Magnetic Dipole Moment and Magnetization 500
A.3 Scalars, Vectors, and Tensors 501
A.3.1 Properties of Matrices 504
Solutions 507
Index 525
Simon Jurt has been working for more than ten years in the NMR department of the University of Zurich. In addition to maintenance and trouble shooting of the NMR spectrometers, he introduces the students to the secrets of NMR spectroscopy, teaches practical NMR courses and is involved in several research projects. His main interests are the experimental NMR techniques, which allow obtaining a plethora of chemo-physical information from the spin physics.
Related Products
-
A Practical Guide to Assay Devel...
13,464.00
16,830.00 -
A Textbook of Modern Toxicology,...
8,942.00
10,520.00 -
Active Pharmaceutical Ingredient...
3,200.00
3,495.00 -
Active Pharmaceutical Ingredient...
14,850.00
17,500.00 -
Advanced Distillation Technologi...
13,500.00
15,000.00 -
Aesthetics Exposed: Mastering Sk...
17,000.00
- Architecture & Construction Management Books
- Biochemistry, Bioengineering and Biotechnology Books
- Books on Analytical Techniques, GC MS, LC, TLC, HPLC, NMR Spectroscopy
- Books on Water and Wastewater Treatment, Analysis Water Treatment Plants
- Chemical Technology, Organic Chemistry, Chemical Synthesis and Chemical Analysis Books
- Cosmetics Science, Cosmetics Formulations, Manufacture and their Analysis Books
- Drugs and Pharma Science Books
- Essential Oils, their Analysis, Natural Products Extraction, Distillation , Isolates Related Books
- Flavor Science, Flavor Analysis, Flavor Creation, Food Flavors Books
- Food Science and Technology, Baking , Food Ingredients , Food Quality , Food Regulations
- Formulations of Perfumes for Agarbatti, Air Freshners, Soaps & Detergents, Fabric Softeners , French style Perfumes, Attars , OUD, Fine Fragrances
- Fragrances, Scents, Attars , Perfumery and Aroma Chemicals Books
- Herbal, Ayurvedic, Medicinal Plants and Aromatic Plants Books
- Law Books
- Major Reference Works (MRWS) Sets - MultiVolume Sets
- Medical Books
- Medical Journals Wolters Kluwer | Medknow India
- NEW ARRIVALS
- Perfumer's Resources
- Special Indian Reprint !
- VILLAGE COTTAGE : Scents and Soaps - Luxury Bathing Soaps
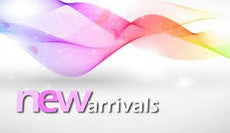
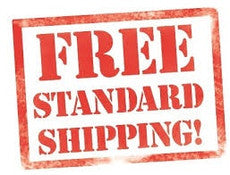